Team:Calgary Entrepreneurial/Project/Technology/
From 2013.igem.org
Emily Hicks (Talk | contribs) |
|||
Line 27: | Line 27: | ||
<html> | <html> | ||
<h1>Developing our Technology</h1> | <h1>Developing our Technology</h1> | ||
- | <p>We are pioneering a live-cell Field-Ready Electrochemical Detection system for toxins present in our environment. Initially, we are focusing on detecting general toxins in oil sands environments, however the immense flexibility our system provides significant opportunities to | + | <p>We are pioneering a live-cell Field-Ready Electrochemical Detection system for toxins present in our environment. Initially, we are focusing on detecting general toxins in oil sands environments, however the immense flexibility our system provides significant opportunities to expand into sensors for almost any compound of interest. With a quick and robust output, our technology offers many advantages over existing biosensor technologies. </p> |
</html> | </html> | ||
Revision as of 07:18, 27 October 2013



FREDsense's website works best with Javascript enabled, especially on mobile devices. Please enable Javascript for optimal viewing.
Developing our Technology
We are pioneering a live-cell Field-Ready Electrochemical Detection system for toxins present in our environment. Initially, we are focusing on detecting general toxins in oil sands environments, however the immense flexibility our system provides significant opportunities to expand into sensors for almost any compound of interest. With a quick and robust output, our technology offers many advantages over existing biosensor technologies.
Overview
Our product is a sensor for a variety of oil and gas related contaminants in water. The system is rapid and portable, allowing for monitoring of toxins much more quickly and easily than present systems allow. Our sensor utilizes a strain of soil bacteria which has been genetically altered to react to toxins in the environment. When the bacteria encounter the toxins they begin producing enzymes capable of changing compounds that can be detected electrochemically. Detection of electroactive chemicals is quantitative and software analysis of the results permits the user to measure the amount of toxin using a simple computer, USB, WiFi, or GPS based interface.
At this point in product development, we have generate a microbial strain that is able to detect three relevant oil sands toxins: naphthenic acids, carbazole, and dibenzothiophene. Further tests have shown that this strain does not respond to an innocuous compound with structural similarity to naphthenic acid or to a general stress inducing compound. We have demonstrated the output of the detector as being able to alter specific compounds so that they may be detected electrochemically. Furthermore we are working to demonstrate that we can detect simultaneously active output signals, where more than one compound can be detected at a time. We currently have a proof-of-concept technology for detecting oil sands toxins.
As we are refining the techniques to optimally detect toxins with the microbial strain, we will be developing a prototype of the system that will be sold commercially and will be ultimately be used by clients for field tests. On this front we are working closely with manufacturers to design our final product.
Description of the Technology
Sensing Microorganism:
To obtain an organism that may respond to petroleum toxins, we performed a transposon screen. This biological screen inserts the genetic sequence of a reporting protein (the enzyme lacZ) into random locations of the test organism's DNA. The purpose is to put the reporter in a region that the host organism uses to survive the toxicity of petroleum toxins because this way it would "tell us" that those chemicals are present.
We performed the transposon screen with the organism Pseudomonas fluorescens Pf-5: a native tailings pond bacteria shown to degrade oil sands toxins. From this screen strains which responded to general oil sands toxins (sulfur-heterocycles, nitrogen heterocycles, and naphthenic acids) were kept for further analysis. We characterized these strains and selected from the original responders, strains that reacted to oil sands toxins but not general hydrocarbon compounds and stress agents (Figure 2).
This response consists of production of a reporter enzyme capable of cleaving a substrate which could then give an electrochemical response. Further testing on one strain using tailings pond samples showed a higher than baseline response, and the organism was capable of survival in the toxic substrate (Figure 3).
Future directions for strain development include identifying and characterizing the genetic sequence of the sensing elements.
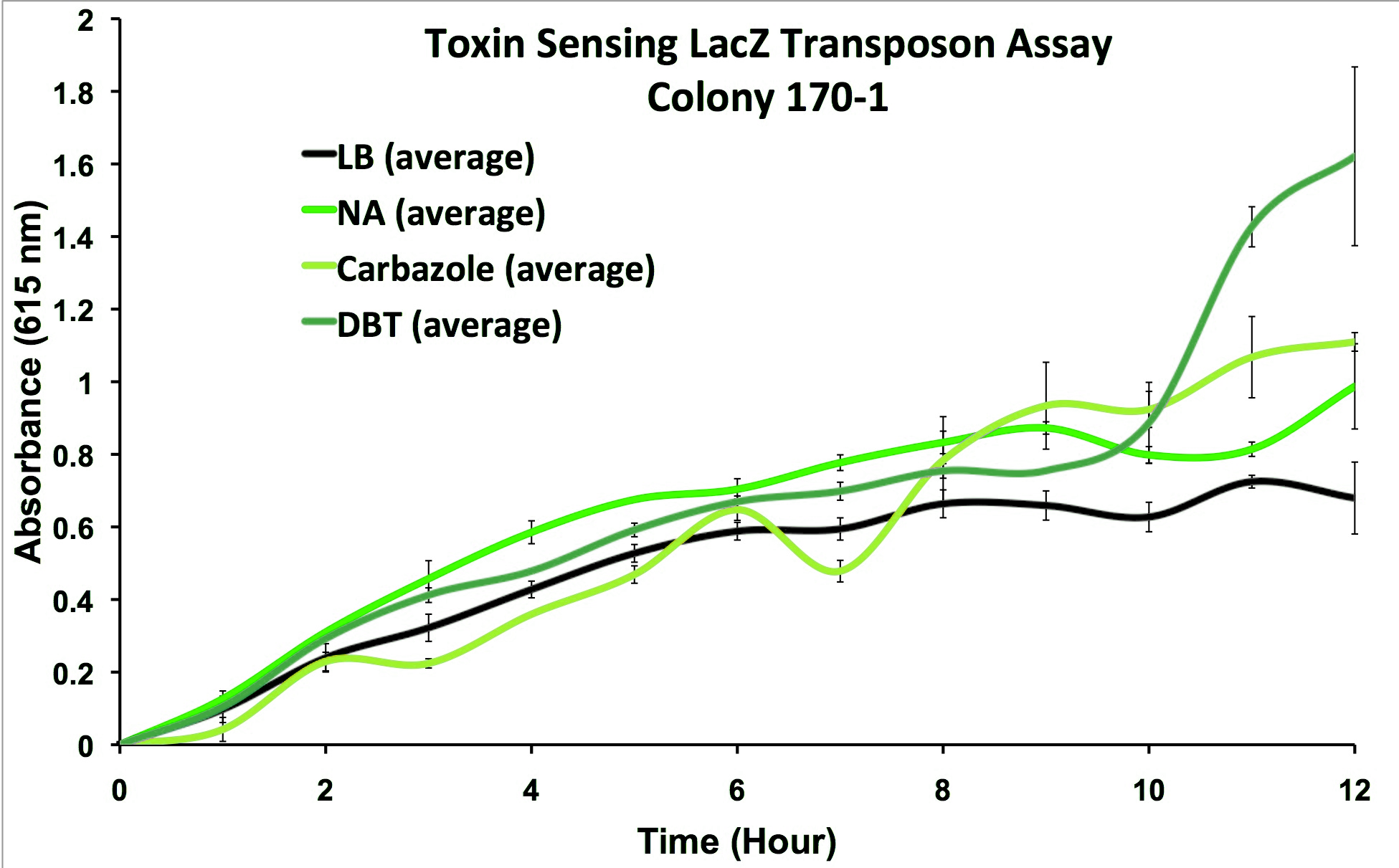
Electrochemical Enzymatic Reporting System:
The enzymes utilized by FREDsense are part of a class of enzymes known as hydrolases. Characteristic of these enzymes is recognition and cleavage of their target structure from another compound that it is bound to. An example of this is the protein β-galactosidase (encoded by the gene lacZ). β-galactosidase recognizes and cleaves galactose bound to other compounds and has traditionally been used for colorimetric reactions in biological science. For our purposes we are using galactose bound to the electroactive compound or analyte CPR-β-D-galactopyranoside (CPRG). Freeing the CPR unmasks its charge, permitting electrochemical detection of it in solution by cyclic voltammetry.
Also characteristic of many hydrolases is the specific recognition of their substrates. The oxidation potential chlorophenol red (CPR), para-diphenol (PDP), and para-nitrophenol (PNP) as substrates was tested. These compounds are conjugated with their sugars to form CPR-β-D-galactopyranoside (CPRG), PDP-β-D-glucopyranoside (PDPG), and PNP-β-D-glucuronide (PNPG). It was found that when measured using cyclic voltammetry, the oxidation potentials of the three substrates were distinct and could be visualised separately on the resulting graph, showing the potential for multiplexing the system.

Hardware, Software, and Prototype Design:
A kinetic model was built for UidA in MatLab, predicting that a response could be detected within 4 minutes of adding the substrate to the enzyme when read electrochemically. This experiment was then performed in the wetlab by inducing UidA expression, and results were consistent with the models prediction.
As well, an inexpensive, small, and portable potentiostat was designed and developed, with required circuitry being printed onto a small circuit board. While larger versions of the potentiostat were shown to function well, an error in the design of the circuit board renders the latest prototype nonfunctional at the moment, preventing further testing and refinement from being done. A custom software package within the MatLab platform was also developed in order to interpret and display the data obtained from electrochemical measurement.
Finally, a prototype device was designed, consisting of a small can-sized container where circuitry would be housed, a slot where a cartridge could be inserted into the housing, and a cartridge where the electrodes, analytes, and microorganisms could be housed with a lid allowing sample injection. The device contained LED lights in order to indicate what step of the reading was currently running as well as to indicate when analysis was finished.
Technical Implementation Plan
In order to realize our first generation product, there are several key steps that need to be taken on the research and development front. As such, we have created a detailed Implementation plan describing these necessary milestones. A condensed version can be seen below. Here we list the major technical milestones that need to be completed. These points are listed in order of priority with the earliest described experiments being most critical in evaluating the commercial potential of our technology. As such, the order represents the relative risk of each experiment, with the most high-risk experiments first, where failure to achieve good results could significantly impact the time and cost estimates for the research and development.
- Final characterization of our first generation sensing organism.
- Transferring our electrochemical system into P. fluorescencs.
- Transitioning our first product into the final prototyping stages.
- Further screening for future generation products.
- Further multiplexing of our electrochemical system.
Responding to Technological Change
The current technology has been carefully designed in order to easily facilitate the production of next generation products. FREDsense’s unique sensing platform is easily adaptable to monitor a plethora of different compounds of interest using different engineered bacterial strains. Second generation products are being developed including detector products specific to unique classes of chemicals including naphthenic acids and hydrocarbons. As these are specific compounds of interest for many oil sands-related processes, detectors capable of monitoring them with specificity are sought-after. These detectors will make use of FREDsense’s novel generation one sensing platform coupled with newly developed bacterial strains. Being able to directly build upon the existing platform will help ensure FREDsense’s new products can be designed quickly and efficiently so as to have a quicker entry to market than generation one products.
With the introduction of more specific and diverse sensors, this will lead the way for multiple-output detector systems. These will include detectors that can monitor multiple different classes of compounds such as combinations of naphthenic acids, hydrocarbons and general toxins simultaneously. As many locations would require monitoring of a variety of compounds of interest at one sampling time, these second generation sensors will allow one water sample to be taken in order to monitor that sample for several toxins. This will allow for quicker sample extraction and faster results. Again, these products will make use of the generation one electrochemical sensing platform with new engineered bacterial strains.
As with generation one, all later generation products will include integrated controls as well as the speed and reliability of the generation one sensing platform. The modular design of FREDsense’s technology will allow the company to adapt quickly and easily to changes in the regulatory landscape in the oil sands market as well as expand into different markets. This will allow FREDsense to expand the breadth of its detection products without investing significant resources into research and development.
Regulatory Hurdles
Biotechnology products in Canada are regulated by three federal agencies: Health Canada for foods, Canadian Food Inspection Agency (CFIA) for seeds and livestock feed, and Environment Canada for new substances intended for environmental release. Our product thus falls under regulation set out by the later. This regulation falls under the Canadian Environmental Protection Act (jointly administered by Environment Canada and Health Canada). Environmental Technology Verification is also required for our technology to be used in this industry. Risk is assessed based on relative toxicity as well as relative amount of exposure to the environment. Specific products are assessed on a one-to-one basis through a dialogue with Environment Canada and Health Canada.