Team:TU-Eindhoven/MRIProcessing
From 2013.igem.org
(→Processing) |
(→MRI Data Processing) |
||
Line 9: | Line 9: | ||
{{:Team:TU-Eindhoven/Template:FloatEnd | caption=a) and b) Solute protons are saturated at their specific resonance frequency in the proton spectrum, this saturation is then transferred to water. c) Measurement of normalized water saturation, the Z-spectrum. d) result of the asymmetry analysis of the Z-spectrum. {{:Team:TU-Eindhoven/Template:RefAgain | id=vanZijlCEST }} | id=CESTExplaination }} | {{:Team:TU-Eindhoven/Template:FloatEnd | caption=a) and b) Solute protons are saturated at their specific resonance frequency in the proton spectrum, this saturation is then transferred to water. c) Measurement of normalized water saturation, the Z-spectrum. d) result of the asymmetry analysis of the Z-spectrum. {{:Team:TU-Eindhoven/Template:RefAgain | id=vanZijlCEST }} | id=CESTExplaination }} | ||
This new type of MRI goes by the name Chemical Exchange Saturation Transfer MRI or CEST MRI{{:Team:TU-Eindhoven/Template:Ref | id=vanZijlCEST | author=van Zijl, Peter, and Nirbhay N. Yadav. | title=Chemical exchange saturation transfer (CEST): what is in a name and what isn't?. | journal=Magnetic Resonance in Medicine | edition=65.4 | pages=8927-948 | year=2011 }}{{:Team:TU-Eindhoven/Template:Ref | id=LiuCEST | author=Liu, Guanshu, et al. | title=Nuts and bolts of chemical exchange saturation transfer MRI. | journal=NMR in Biomedicine | edition= | pages= | year=2013 }}{{:Team:TU-Eindhoven/Template:Ref | id=GiladGene | author=Gilad, Assaf A., et al. | title=MRI reporter genes. | journal=Journal of Nuclear Medicine | edition=49.12 | pages=1905-1908 | year=2008 }}. The principle behind this technique is based on compounds that contain pools of exchangeable protons that can be selectively saturated using radiofrequency irradiation. Upon proton exchange with bulk water, these compounds can be indirectly visualized by measuring the bulk water. The amino acids Lysine, Arginine, Threonine and Serine contain those exchangeable protons and polypeptides containing those amino acids in abundance are therefore potential contrast agents {{:Team:TU-Eindhoven/Template:Ref | id=GoffeneyCEST | author=Goffeney, Nicholas, et al. | title=Sensitive NMR detection of cationic-polymer-based gene delivery systems using saturation transfer via proton exchange. | journal=Journal of the American Chemical Society | edition=123.35 | pages=8628-8629 | year=2001 }} {{:Team:TU-Eindhoven/Template:Ref | id=GiladCEST | author=Gilad, Assaf A., et al. | title=Artificial reporter gene providing MRI contrast based on proton exchange. | journal=Nature biotechnology | edition=25.2 | pages=217-219 | year=2007 }}. Another advantage of CEST MRI is that different kinds of protons (e.g. amide and hydroxide protons) can be distinguished, which allows for 'multi-color' imaging when using multiple contrast agents simultaneously {{:Team:TU-Eindhoven/Template:Ref | id=McMahonMulticolor | author=McMahon, Michael T., et al. | title=New 'multicolor' polypeptide diamagnetic chemical exchange saturation transfer (DIACEST) contrast agents for MRI. | journal=Magnetic Resonance in Medicine | edition=60.4 | pages=803-812 | year=2008 }}. | This new type of MRI goes by the name Chemical Exchange Saturation Transfer MRI or CEST MRI{{:Team:TU-Eindhoven/Template:Ref | id=vanZijlCEST | author=van Zijl, Peter, and Nirbhay N. Yadav. | title=Chemical exchange saturation transfer (CEST): what is in a name and what isn't?. | journal=Magnetic Resonance in Medicine | edition=65.4 | pages=8927-948 | year=2011 }}{{:Team:TU-Eindhoven/Template:Ref | id=LiuCEST | author=Liu, Guanshu, et al. | title=Nuts and bolts of chemical exchange saturation transfer MRI. | journal=NMR in Biomedicine | edition= | pages= | year=2013 }}{{:Team:TU-Eindhoven/Template:Ref | id=GiladGene | author=Gilad, Assaf A., et al. | title=MRI reporter genes. | journal=Journal of Nuclear Medicine | edition=49.12 | pages=1905-1908 | year=2008 }}. The principle behind this technique is based on compounds that contain pools of exchangeable protons that can be selectively saturated using radiofrequency irradiation. Upon proton exchange with bulk water, these compounds can be indirectly visualized by measuring the bulk water. The amino acids Lysine, Arginine, Threonine and Serine contain those exchangeable protons and polypeptides containing those amino acids in abundance are therefore potential contrast agents {{:Team:TU-Eindhoven/Template:Ref | id=GoffeneyCEST | author=Goffeney, Nicholas, et al. | title=Sensitive NMR detection of cationic-polymer-based gene delivery systems using saturation transfer via proton exchange. | journal=Journal of the American Chemical Society | edition=123.35 | pages=8628-8629 | year=2001 }} {{:Team:TU-Eindhoven/Template:Ref | id=GiladCEST | author=Gilad, Assaf A., et al. | title=Artificial reporter gene providing MRI contrast based on proton exchange. | journal=Nature biotechnology | edition=25.2 | pages=217-219 | year=2007 }}. Another advantage of CEST MRI is that different kinds of protons (e.g. amide and hydroxide protons) can be distinguished, which allows for 'multi-color' imaging when using multiple contrast agents simultaneously {{:Team:TU-Eindhoven/Template:Ref | id=McMahonMulticolor | author=McMahon, Michael T., et al. | title=New 'multicolor' polypeptide diamagnetic chemical exchange saturation transfer (DIACEST) contrast agents for MRI. | journal=Magnetic Resonance in Medicine | edition=60.4 | pages=803-812 | year=2008 }}. | ||
+ | |||
+ | ==Methods== | ||
+ | This theory can be used to scan our library of potential contrast agents ([[Team:TU-Eindhoven/Production | Protein Selection]]). Hereto the proteins were (aerobically) overexpressed in BL21 using a pET28a vector with a T7 promotor. The bacteria were spun down and fixed in PFA. The whole pellet was then measured in a 7 T Bruker MRI machine. First, the correct water frequency was determined, the machine was shimmed, i.e. a homogeneous magnetic field was created. The first measurement was a T<sub>2</sub> weighed image for general orientation. Subsequentlylocal shimming was performed on each of the separate pellets. For the final measurements, the saturation pulse was set to vary from $\pm -4 ppm$ to $\pm +4 ppm$ (relative to water), the measurements were averaged over 8 separate scans. Also a $S_{0}$ (without saturation pulse) image was taken. | ||
==Processing== | ==Processing== | ||
Line 35: | Line 38: | ||
</html> | </html> | ||
{{:Team:TU-Eindhoven/Template:FloatEnd | caption=Comparison of agent samples and the control sample. | id=ContrastComparison }} | {{:Team:TU-Eindhoven/Template:FloatEnd | caption=Comparison of agent samples and the control sample. | id=ContrastComparison }} | ||
- | |||
- | |||
- | |||
==Results== | ==Results== |
Revision as of 09:20, 4 October 2013



Contents |
MRI Data Processing
Until recently, the most common way of generating MRI contrast was to use heavy metal based contrast agents. Examples of these are the commercially available Gadolinium(III) based Magnevist and the Iron Oxide based Resovist. An obvious downside of using heavy metals is the toxicity of the agents in the human body. Fortunately, a new type of MRI has been developed, which allows for the use of organic compounds as contrast agent.
The Principle
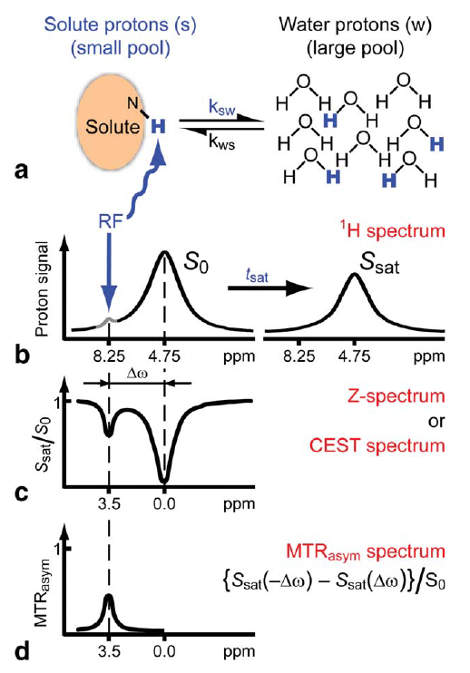
Methods
This theory can be used to scan our library of potential contrast agents ( Protein Selection). Hereto the proteins were (aerobically) overexpressed in BL21 using a pET28a vector with a T7 promotor. The bacteria were spun down and fixed in PFA. The whole pellet was then measured in a 7 T Bruker MRI machine. First, the correct water frequency was determined, the machine was shimmed, i.e. a homogeneous magnetic field was created. The first measurement was a T2 weighed image for general orientation. Subsequentlylocal shimming was performed on each of the separate pellets. For the final measurements, the saturation pulse was set to vary from $\pm -4 ppm$ to $\pm +4 ppm$ (relative to water), the measurements were averaged over 8 separate scans. Also a $S_{0}$ (without saturation pulse) image was taken.
Processing
To detect these protons in bulk water some processing steps are required. First of all, the imaging should be taken at different frequencies of the saturation pulse. This way the signal at different chemical shifts can be measured. When bulk water is set to 0 ppm, the signal plot will be roughly symmetric around zero, since saturation pulses will excite the bulk water in a similar way when the absolute shift is equal. This also implies that when the signals with a negative shift from the bulk water are compared with the signals with a positive shift, asymmetry can be detected. The CEST effect is precisely what causes this asymmetry in the signal plot, due to the chemical shift of the CEST compounds being different from the chemical shift of water. Therefore, when the absolute shift from the bulk water of two saturation pulses is equal, this does not mean the absolute shift from the CEST compound is equal and the CEST protons will in one case hardly saturate at all (depending on its shift from bulk water of course).
Putting this principle into practice requires some processing steps. First of all, the asymmetric comparison should be conducted as shown in . Where the MTRasym is the asymmetry in the z-spectrum. Furthermore, $S_{0}$ and $S_{w}^{\pm\Delta\omega_{sw}}$ are, respectively, the water signals without saturation and with saturation at $\Delta\omega_{sw}$ from bulk water. The PTE is the Proton Transfer Enhancement, which gives an indication of the sensitivity of an agent, independent of the concentration. In this research, the concentration was not measured accurately, but the mass of cell pellet was aimed to be kept constant, so the MTRasym plots can still be compared quite well.
To eliminate the background signal of other Lysine and Arginine rich proteins, the asymmetric plots of the potential agents were compared to the asymmetric plots of a control sample, where no protein was over-expressed. In practice this can be compared to using an MRI image of human tissue without contrast agent as control for an image of tissue with contrast agent. This comparison was done in two different ways, one option results in the absolute difference compared to the control sample. The other method is relatively comparing the samples ().
Results
When the previously described processing steps are applied to the MRI results of this research, the following graphs can be acquired. shows the MTRasym, $C_{abs}$ (Absolute Difference) and $C_{rel}$ (Relative Difference) graphs for all potential contrast agents that were tested.
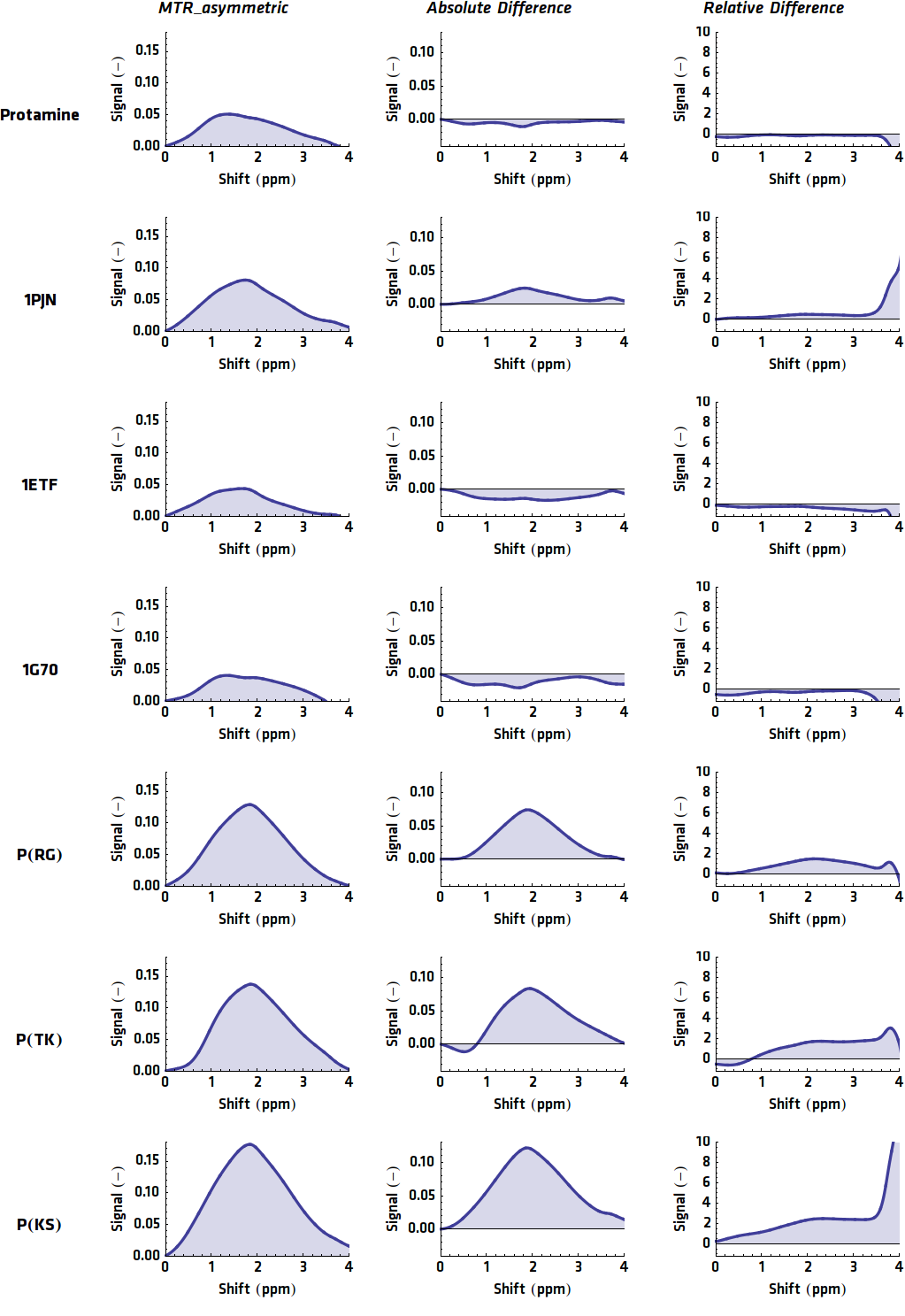
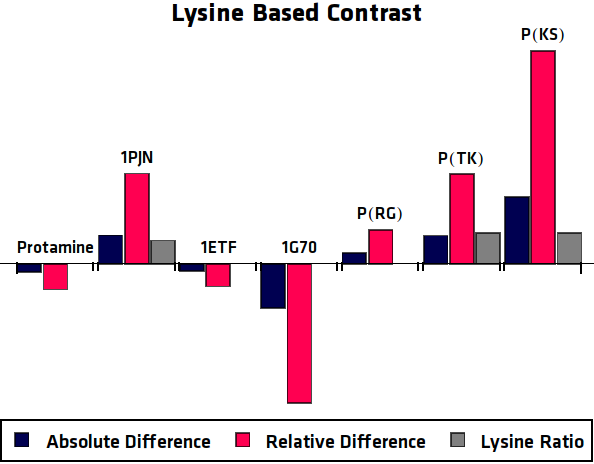
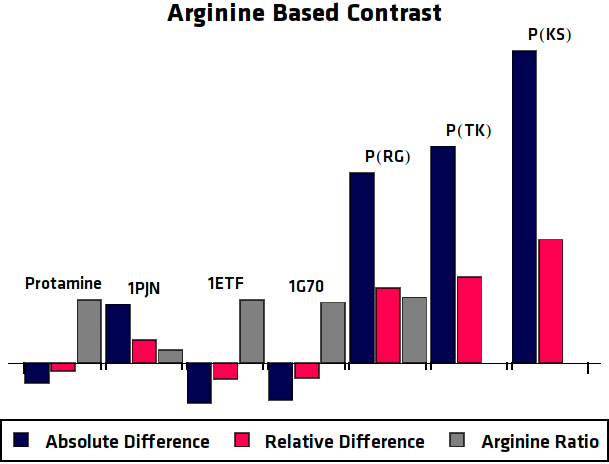
Conclusion
All in all, the most promising candidate agent seems to be 1PJN. The lysine peak of this agent is very clearly distinguishable from the rest of the signal. As a arginine based compound, no clear results have been achieved and therefore no good recommendation can be given, except that the arginine based agents are less suitable for generating contrast in general. Therefore, it is recommended to use lysine based agents, such as 1PJN, only for multi-color MRI or imaging lysine rich areas arginine agents can be useful. Another conclusion that can be drawn from the MRI results, is that Poly(KS) has actually been expressed in the bacteria. This is contradictory to the other lab results, where no traces of Poly(KS) on SDS-Page gels were found. The most obvious explanation for these results, is that only a very small part of the Poly(KS) chain was created, which is to be expected due to the largely repetitive and non-optimal DNA of the construct.
References